Superconductivity temperatures on the rise
- Mateo Cardinal
- Nov 28, 2023
- 10 min read
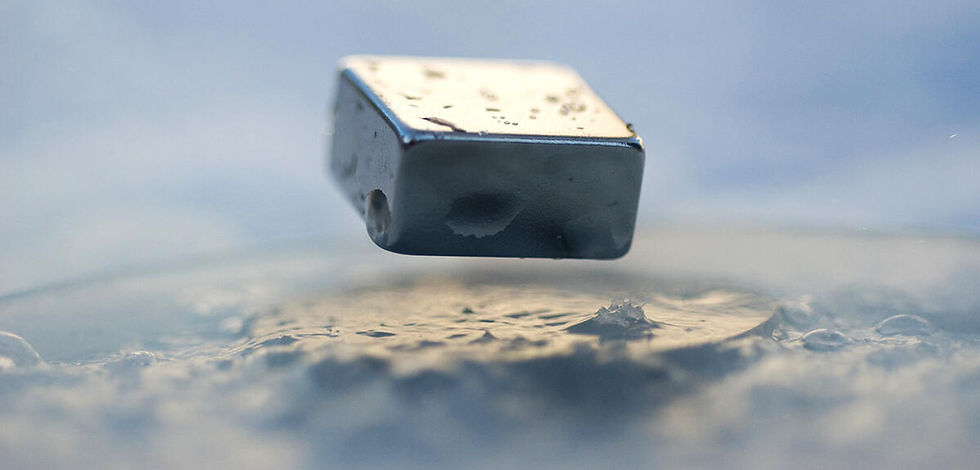
Superconductivity is the property of certain materials that can conduct electric currents with no resistance. This quantum phenomenon is still shrouded in mystery, and until now has been limited to very low temperatures. Yet all this could soon change.
Imagine a world in which currents circulate with no loss in high-voltage lines, electric appliances and electronic devices consume very little energy, and trains travel at very high speeds levitating above rails, with no friction. Superconductivity could one day make these tantalising prospects become reality.
This physical phenomenon, discovered over a century ago, is observed in certain materials through the disappearance of electrical resistance, and hence of any energy loss. This unique property has already enabled the development of key applications, such as magnetic resonance imaging (MRI) in hospitals, and particle accelerators in physics research. Unfortunately, to acquire this extraordinary capacity, most of the superconducting materials developed to date must be cooled to extreme temperatures near absolute zero (- 273.15 °C) by expensive and cumbersome refrigeration systems, thereby limiting their use to a few niche applications.

But researchers have not said their last word, for in laboratories across the globe, they are trying to shed light on the mechanisms behind superconductivity by designing and closely studying new, promising materials. Their sights are set on discovering room-temperature superconductors that require no refrigeration, which could revolutionise our daily lives.
From the strange phenomenon observed…
This state of matter was discovered in 1911, when the Dutch physicist Heike Kamerlingh Onnes found that mercury’s electrical resistance disappeared when it was cooled to - 269°C using liquid helium. This is a surprising characteristic, because under normal conditions even the most conductive electrical wires lose some of their energy in the form of heat. Many other superconducting metals (lead, tin, aluminium) and metal alloys have been discovered since, but always at temperatures approaching absolute zero.
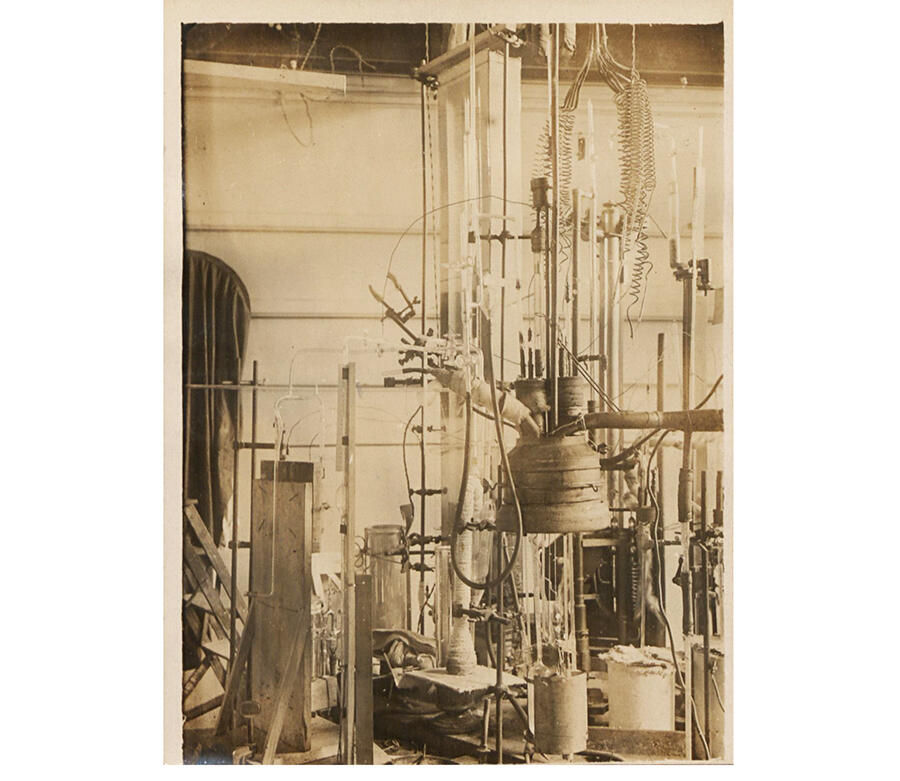
It was not until the 1950s – 1957 to be precise – that this phenomenon was finally explained by three American physicists who proposed the BCS theory, named after the initials of their respective names: John Bardeen, Leon Neil Cooper, and John Robert Schrieffer.
Superconductivity originates in the behaviour of the electrons that make up atoms; understanding the phenomenon requires quantum physics, which describes matter on that scale. “It is one the rare manifestations on the macroscopic scale of a quantum physics effect,” points out Cyril Proust of the CNRS’s LNCMI laboratory. Indeed, as the temperature falls in the material, the movement of atoms decreases until they generate particular vibrations within the crystalline structure, causing the electrons to form pairs and then combine together, ultimately forming a collective wave throughout the material.
…to early successes
From that point forward nothing disturbs the movement of the electrons, resulting in the disappearance of electrical resistance. However, the phenomenon vanishes above a particular “critical” temperature, with thermal agitation destroying this fragile balance. In addition to the loss of electrical resistance, research over the years has revealed another striking property: a superconductor expulses any magnetic field imposed on it. This is known as the Meissner effect, which was named after its discoverer. It is therefore no wonder that superconductivity quickly found applications. All it takes is inject a high-intensity current in a superconducting coil for it to generate an equally intense magnetic field, with no risk of overheating. And it is also possible to literally levitate a magnet simply by positioning it above a superconductor.
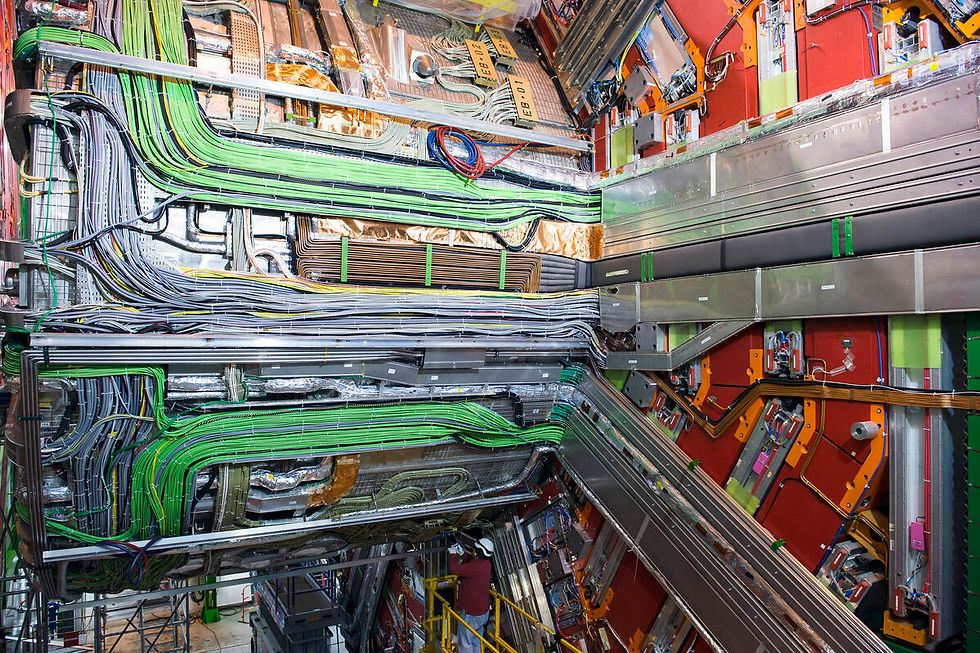
These intense magnetic fields are, among other things, indispensable to the MRI machines that analyse the bodily tissue of patients; the magnetic resonance spectroscopy used by scientists to reveal a sample’s molecular structure; experimental nuclear fusion reactors such as ITER, which is currently being built in France; and to the particle accelerators in which giant superconducting magnets deflect and focus particle beams.
With that in mind, if the LHC at CERN in Geneva were to use conventional copper magnets, it would measure 100 kilometres in circumference rather than 27, and would consume nearly 25 times the energy! In other words, it would never have seen the light of day. Despite this fine early success, scientists have remained firmly grounded. According to the BCS theory, which clearly identifies the mechanism at work, they know that in order to reach superconductivity, a metal must be cooled to a very low temperature. Very few imagined that they could one day achieve this at a higher temperature, let alone room temperature!
An unexpected discovery
But a discovery completely changed everything. In 1986, the first superconductors with a “high critical temperature” were unexpectedly identified, at temperatures higher than those previously observed. These synthetic materials, which are compounds based on copper oxide, are known as cuprates. Today the record temperature for reaching the superconducting state held by a (mercury-based) cuprate is - 135°C. This is still cold, but much more attainable thanks to liquid nitrogen. This finding sparked new hope in the scientific community that superconductivity could exist at room temperature.
Researchers strove to decipher this new type of superconductivity exhibited by cuprates, with a view to improving it and eventually finding room-temperature superconductors. “Experiments quickly showed that we were dealing with a non-conventional superconductivity. As with metals, it is the pairing of electrons that creates the phenomenon, but contrary to what is described in the BCS theory, it is not the vibration of atoms that serves as the glue between electrons,” Proust explains.
The mystery of cuprates
Elucidating the nature of this mysterious glue has become the main goal, and even represents one of the primary research subjects in condensed matter physics. To make progress, theorists and experimenters are working together to considerably improve measurement techniques for materials, and to invent new theoretical concepts as well as digital approaches to describe them. In thirty years, this research has allowed many advances in the field of superconductivity, and more broadly that of physics.
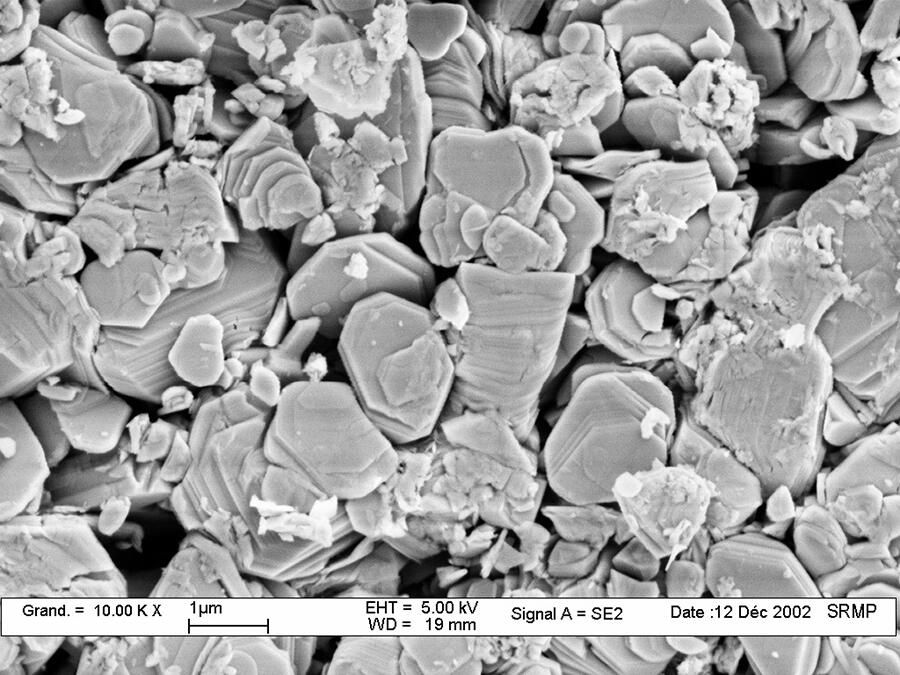
“It must be acknowledged that the mystery of high-temperature superconductivity has not yet been solved. Multiple explanations for its origin have been proposed, but none has garnered consensus,” admits Alain Pautra from the CRISMAT. This remains an open question because cuprates are puzzling materials with complex physics, even when they are not superconductors. Initially, a cuprate is a totally insulating material; it is only by adding or removing electrons from it via a chemical modification known as “doping” that it transforms into an electrical conductor, and then into a superconductor at lower temperatures.
Group effect
Unlike a metal, in which electrons can be considered independent, in a cuprate they are “highly correlated”: they get in each other’s way, block one another, and move only collectively, which makes them extremely difficult to describe – and their behaviour impossible to understand. To complicate matters further, researchers also know that in the insulating state, a cuprate is a magnetic material, such that today there are two dominant scenarios in trying to explain the superconductivity of these copper oxides.
Some believe that it is this same magnetism – or rather small magnetic fluctuations that remain in trace amounts near the critical temperature – that provides the “glue” for electron pairs. “Numerous observations strongly suggest this, although no direct proof has yet been established,” reveals Proust, who supports this theory. Others emphasise that, under the effect of these strong correlations, electrons organise in various configurations or orders, each more exotic than the other.
These different configurations could compete with one another, with the resulting emergence of superconductivity. “Experiments have helped to clearly characterise these electronic orders, although their diversity makes it difficult to demonstrate a cause and effect connection with superconductivity,” admits Pautrat.
An experimental challenge
Researchers are still awaiting the final experiment that will settle the debate. Proust, who has already helped to advance the field, intends to play his part. Since 2003, he has exposed cuprate samples to very intense magnetic pulses that suppress their superconductivity. “In doing so, we reveal the properties the material would have without establishing a superconducting phase. This allowed us to shine a light on these competing electronic orders.”

Going even further, Proust and his team are preparing their next experiment, in which cuprates will be exposed to unprecedented magnetic fields of 200 teslas (or 2,000 times more powerful than the magnet stuck to your refrigerator), the only way to destroy superconductivity when the critical temperature is at its maximum, and the phenomenon is at its most intense. “The experimental challenge is considerable, but it is one of the keys to solving this mystery.”
New classes of materials
The study of other superconducting materials with high critical temperatures could also contribute to discoveries, for while cuprates are stars in terms of temperature records, they are not the only representatives of this new class of superconductors. In 2008, scientists evidenced the superconductivity of pnictides, iron-based compounds whose transition temperature towards the superconducting phase is - 217°C. Less complex to describe than their copper oxide-based cousins, pnictides have revealed that in their case, it is probably magnetism alone that allows electrical charges to form pairs. More recently, in 2018, nickelates, which are based on nickel oxide and have a critical temperature on the order of - 258°C were added to the list.
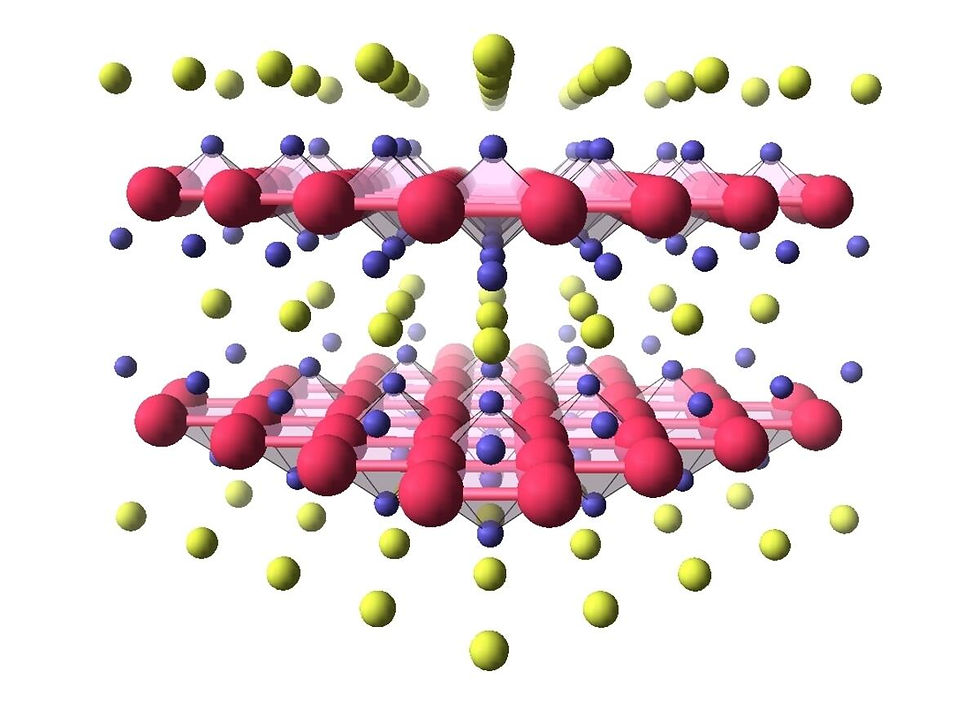
For these compounds that are structurally very similar to cuprates – and that have been considered as potential superconductors for twenty years – it appears that magnetism does not play a role in the appearance of superconductivity, bringing grist to the theorists’ mill. “If we want to understand high-temperature superconductivity one day, it is crucial to compare different families of materials. Only then can we acquire a global view of the phenomenon, and identify the most important mechanisms,” stresses Marie-Aude Méasson of the CNRS’s Institut Néel.
Hydrides: the new prodigy
Control of high-temperature superconductivity represents a small revolution for electrical and electronic networks, but there should be no expectations of sweeping changes as long as reliance on cooling systems remains, as the true revolution can only be achieved at room temperature. Fortunately, superconductivity is full of new developments, for while cuprates have not yet revealed their mysteries, the focus has shifted towards other materials known as hydrides.
Interest in these hydrogen-rich compounds emerged in 2015, when a team of German researchers showed that hydrogen sulfide becomes a superconductor at - 70 °C, a much higher temperature than that of cuprates. However, the flip side is that this record was established under monstrous levels of pressure: to reach a superconducting state, a sample must be exposed to pressure on the order of 2 million bars, or 2 million times that of our atmosphere! Physicists used diamond anvil cells, the hardest known material, to achieve this.
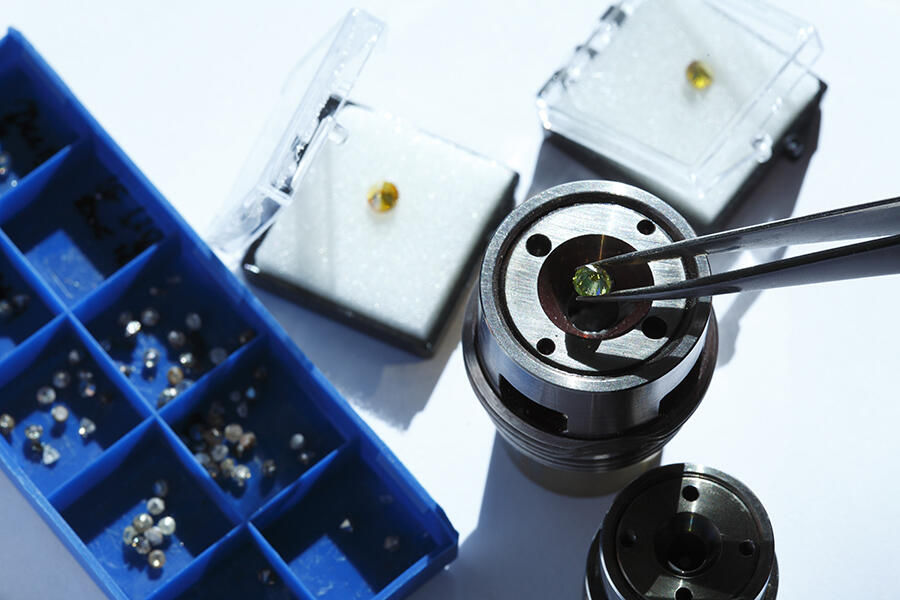
Carrying this out was nevertheless important, as it confirmed for the first time a prediction made in 1969 based on the BCS theory, which identified metallic hydrogen – when exposed to very high pressure – as a candidate for very high-temperature superconductivity. It also encouraged other groups to follow this path, so much so that in recent years, a dozen hydrides have been synthesised.
Increasing announcements and media buzz
Then came an American team’s exceptional announcement last March in the journal Nature regarding a compound made of hydrogen, lutetium, and nitrogen that is a superconductor at 20.5°C under a pressure of “only” 10,000 bars. This result, which was eventually withdrawn by Nature in November 2023 upon the request of its authors, shook up the scientific community, for not only was room temperature achieved, but the pressure applied was much more accessible, with such levels being common in certain industrial processes. “This research is the most spectacular of the last twenty years. Showing that room temperature is an attainable objective totally revitalised the field. But we should remain prudent until the results have been reproduced and confirmed,” observes Pautrat.
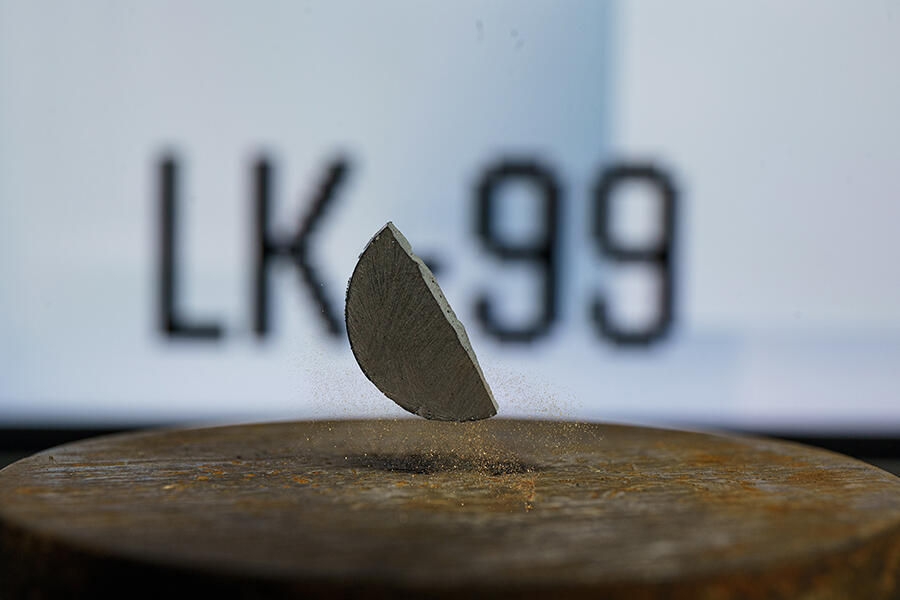
Careful not to claim victory prematurely, numerous teams across the globe immediately focused on this new material in an attempt to synthesise it and confirm the result. “To date none of the groups that reproduced the experiment has observed a superconducting phase. It is all the more disturbing given that the pressure conditions are relatively simple to put in place,” adds Méasson. This cast doubt on the discovery, with criticisms in the scientific community pointing to errors and shortcomings in the publication, especially the fact that the compound’s crystallographic structure – which defines the proportion and 3D organisation of atoms – has not yet been clearly identified. Furthermore, Nature withdrew another controversial article by the same authors published in 2020, which announced the discovery of the first hydride superconductor at 15 °C.
In light of this, some scientists have not hesitated to speak of fraud. The same media buzz and subsequent criticisms tarnished the much sensational announcement this summer of the superconducting properties of the material LK-99 (see text box) attained at room temperature and under ambient pressure.
Promising flaws
Some researchers have proposed a very precise measurement in order to definitively settle the debate, such as Jean-François Roch and his team at the Light, Material, and Interfaces Laboratory. The physicist uses the quantum properties of diamond NV centres, occasional flaws within the crystal that behave like artificial atoms, and that are extremely sensitive to surrounding magnetic fields. “The idea is to implant these NV centres on the tips of the diamond anvils in order to measure the Meissner effect in the material, thereby offering direct proof of its superconductivity. For the measurement of magnetic properties via traditional sensors has proved extremely difficult under such pressure conditions until now. Only NV centres can provide unambiguous proof of this effect,” explains Roch. His method can verify the superconductivity of the most recent hydride, which raises much controversy, as well as that of the other hydrides that are currently the subject of heated debate within the scientific community.
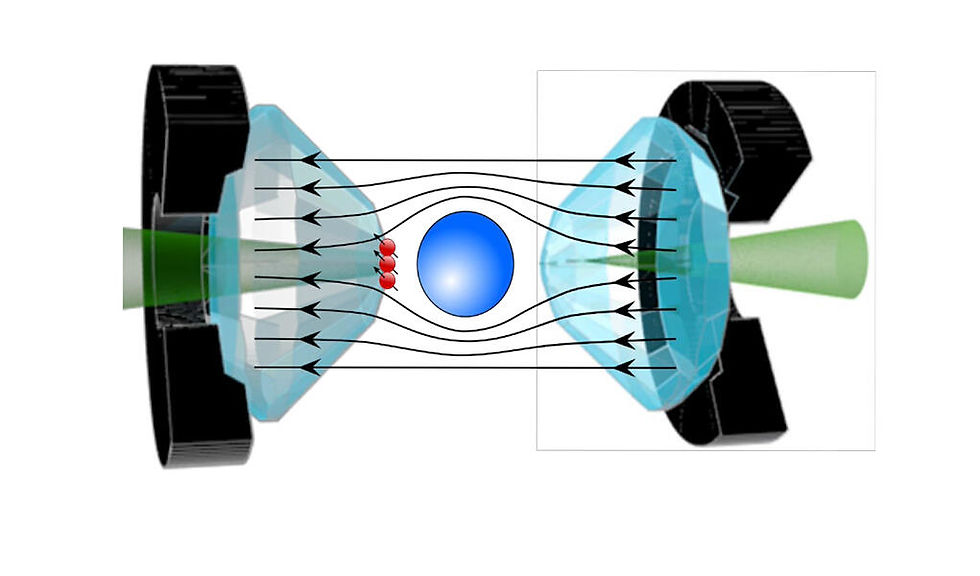
Beyond the controversy, these new compounds represent one of the best hopes for attaining room-temperature superconductivity, although the gargantuan pressure needed for superconductivity remains a genuine stumbling block to their use in everyday life. Some believe that this obstacle is not insurmountable, for just as diamonds formed in the Earth’s mantle retain their properties in the conditions prevailing on the surface, metastable forms of hydrides could be found, which remain superconductors even when the pressure is eased.
Physicists and chemists are well aware of this, and are working hand in hand to discover new superconducting materials through a clever mix of theory, digital simulations, and relentless testing for new combinations of elements. “Alongside theoretical efforts, research on superconductivity is often a matter of intuition, scientific empiricism, and sometimes even chance. Just as the discovery of cuprates was unexpected, a surprise is always possible in the quest for the ultimate superconductor,” concludes Pautrat. The revolution is underway.
LK-99, a rejected candidate
After a first announcement by an American team last March in the journal Nature regarding a hydrogen-based material that is a room-temperature superconductor at a pressure of 10,000 bars, last July it was a Korean team’s turn to announce the development of a superconducting material, this time at room temperature and under ambient pressure. The compound, named LK-99, which consists of phosphorus, copper, and lead – and breaks all temperature and pressure records – is potentially revolutionary. However, like the work of the American researchers, this result must be approached with extreme caution, for it has never been published in a scientific journal, and none of the attempts by other groups to reproduce it has confirmed superconductivity of any kind.
“The characteristic curves are not very convincing: they do not resemble the properties of a superconducting material. I do not see it as promising,” deems Pautrat from the CRISMAT. It is important not to claim victory prematurely, and it is even a safe bet that this result will never be confirmed. In this field where economic stakes are high, announcements of room-temperature superconductors are regularly made – and quickly contradicted. Much more so than buzz, the road to the perfect superconductor calls for patience and scientific integrity. French National Centre for Scientific Research (CNRS)
Comments